Medical milestone: Making blood stem cells in the lab
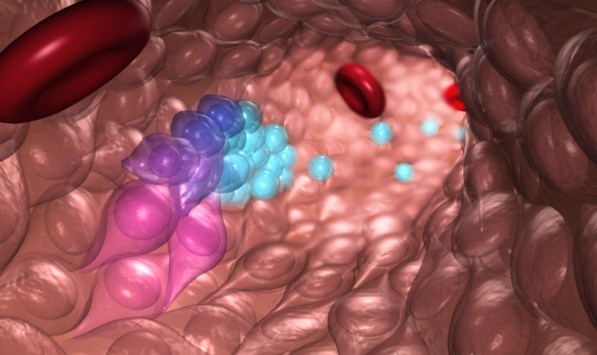
Pluripotent stem cells can make virtually every cell type in the body. But until now, one type has remained elusive: blood stem cells, the source of our entire complement of blood cells.
Since human embryonic stem cells (ES cells) were isolated in 1998, scientists have tried to get them to make blood stem cells. In 2007, the first induced pluripotent stem (iPS) cells were made from human skin cells, and have since been used to generate multiple cell types, such as neurons and heart cells.
But no one has been able to make blood stem cells. A few have have been isolated, but they’re rare and can’t be made in enough numbers to be useful.
Now, the lab of George Daley, MD, PhD, part of Boston Children’s Stem Cell Research program as finally hit upon a way to create blood stem cells in quantity, reported today in Nature.
The cells they made are actually a mix of true blood stem cells and blood progenitor cells. But they were capable of generating multiple types of human blood cells when put into mice.
“We’re tantalizingly close to generating bona-fide human blood stem cells in a dish,” says Daley, who is also dean of Harvard Medical School. “This work is the culmination of over 20 years of striving.”
Making custom blood cells for patients
Ironically, blood stem cell transplant — in the form of a bone-marrow transplant — was the first kind of stem-cell therapy to be used clinically. Bone marrow transplants offer a cure for certain cancers, blood disorders, immune deficiencies and even metabolic disorders. But they require a matched donor. When a perfect match can’t be found, harsh immune-suppressing treatments are needed to prevent dangerous graft-versus-host reactions, in which the donor cells attack the patient’s cells.
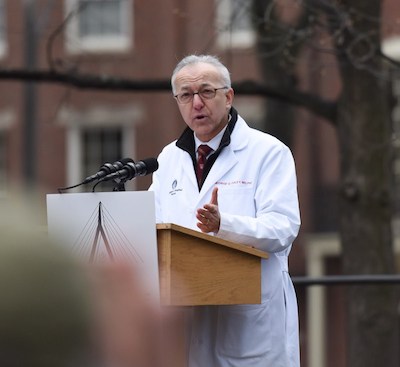
Blood stem cells can also be obtained, more safely, from banked umbilical cord blood. But the number of blood stem cells in a single cord often isn’t enough for older children and adults. The lab of Leonard Zon, MD, also part of Boston Children’s Stem Cell Research Program, has been working for a decade to discover drugs that boost production of blood stem cells.
In 2013, the Daley and Zon labs successfully got iPS cells to make blood progenitor cells. This, too, was a helpful work-around, since these cells also make various kinds of blood cells. It allowed the team to model Diamond Blackfan anemia in a dish and conduct high-throughput drug screening.
But the work published today brings the world even closer to making bona-fide stem cells on demand.
“This step opens up an opportunity to take cells from patients with genetic blood disorders, use gene editing to correct their genetic defect and make functional blood cells,” says Ryohichi (Rio) Sugimura, MD, PhD, the study’s first author and a postdoctoral fellow in the Daley Lab. “This also gives us the potential to have a limitless supply of blood stem cells and blood by taking cells from universal donors. This could potentially augment the blood supply for patients who need transfusions.”
A two-part recipe for blood stem cells
Sugimura, Daley and colleagues combined two approaches. First, they mimicked normal embryonic development by exposing human pluripotent stem cells (both ES and iPS cells) to chemical signals that direct stem cells to differentiate into specialized cells. This generated something called hemogenic endothelium, an early embryonic tissue that eventually gives rise to blood stem cells.
In the second step, the team added transcription factors — genetic regulatory factors that push the hemogenic endothelium toward a blood-forming state. Starting with 26 such factors, they eventually came down to just five (RUNX1, ERG, LCOR, HOXA5 and HOXA9) that were both necessary and sufficient for creating blood stem cells. They delivered the factors into the hemogenic endothelial cells with a lentivirus, a vector sometimes used in gene therapy. Then they transplanted these genetically engineered cells into mice.
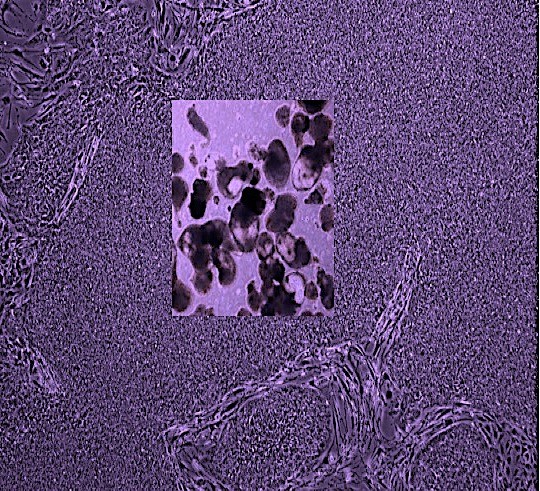
Weeks later, a few of the mice carried multiple types of human blood cells in their bone marrow and blood. These included red blood cell precursors, myeloid cells (precursors of monocytes, macrophages, neutrophils, platelets and other cells), and T and B lymphocytes. Some mice could mount a human immune response after vaccination.
ES cells and iPS cells produced blood stem and progenitor cells about equally well with this technique. But iPS cells are of most interest to the team, since they offer the ability to derive cells directly from patients and model disease.
“We’re now able to model human blood function in so-called ‘humanized mice,’” says Daley. “This is a major step forward for our ability to investigate genetic blood disease.”
What are blood stem cells, anyway?
The researchers ultimately hope to churn out blood stem cells in a dish in a way that’s practical and safe, without using delivery viruses. They also want to introduce gene-editing techniques like CRISPR to correct genetic defects in iPS cells before the blood stem cells are made. The corrected cells could then be returned to patients.
This work is the culmination of over 20 years of striving.”
George Daley
To meet these goals, they’ll likely need to solve a long-standing problem. No one’s been able to define what exactly bona-fide blood stem cells are.
“It’s proved challenging to ‘see’ these cells,” says Sugimura. “It’s been like blind people touching an elephant, trying to see what kind of creature it is. You can roughly characterize blood stem cells based on surface markers. But even with this, it may not be a true blood stem cell. And once it starts to differentiate and make blood cells, you can’t go back and study it. It’s already gone.”
The study was supported by the National Institute of Diabetes and Digestive and Kidney Diseases; the National Institute of Allergy and Infectious Diseases; the National Heart, Lung, Blood Institute; Alex’s Lemonade Stand; the Doris Duke Medical Foundation; the American Society of Hematology and the Howard Hughes Medical Institute.
More in this Nature commentary and news coverage in The Scientist, New Scientist, and the Los Angeles Times. This and a related study were also highlighted in the NIH Director’s Blog.
Related Posts :
-
Microvillus inclusion disease: From organoids to new treatments
Microvillus inclusion disease (MVID) is a rare type of congenital enteropathy in infants that causes devastating diarrhea and an inability ...
-
Blood donations help Kit manage Diamond-Blackfan anemia — so she can dance, sing, and enjoy life
Every month, Kit Murdoch needs a blood transfusion to stay alive. The 2-year-old has Diamond-Blackfan anemia, a rare ...
-
Sickle cell gene therapy and boosting fetal hemoglobin: A 75-year history
Ed. Note: This post updates an earlier post from 2018. In a landmark decision today, the Food and Drug Administration (FDA) ...
-
The clot thickens: Kellie Machlus, PhD
Part of an ongoing series profiling researchers at Boston Children’s Hospital. Platelets are the bandages of our blood, forming ...