Sickle cell gene therapy and boosting fetal hemoglobin: A 75-year history
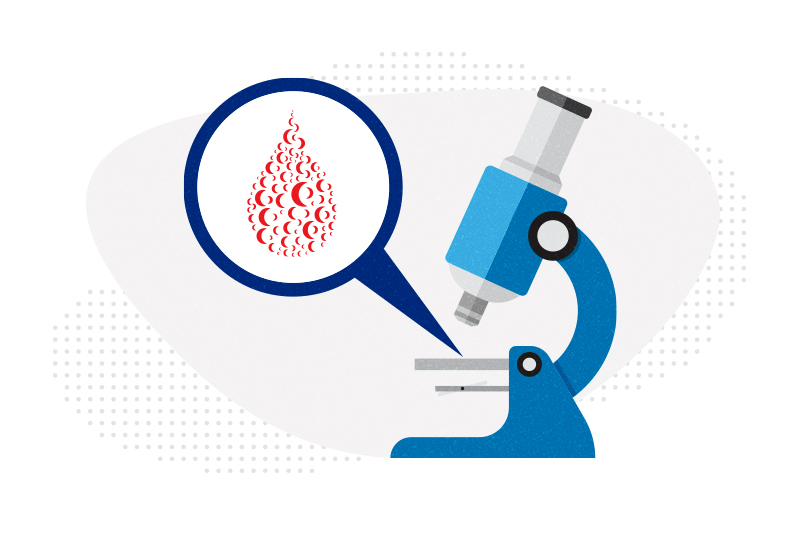
Ed. Note: This post updates an earlier post from 2018.
In a landmark decision today, the Food and Drug Administration (FDA) approved two gene therapies for sickle cell disease. One of them, Casgevy, has deep scientific roots at Boston Children’s Hospital — and is also the first therapy using CRISPR gene editing to gain FDA approval for any condition.
Casgevy works by increasing production of the fetal form of hemoglobin, which people normally stop making after birth, and reducing production of the adult form of hemoglobin. Hopes are high that it could cure sickle cell disease permanently or make it a much milder condition.
Found in all our red blood cells, hemoglobin is responsible for carrying oxygen throughout the body. In sickle cell disease, a mutation causes hemoglobin molecules to form a rigid chain. This causes red blood cells to stiffen into a sickle shape and clog up blood vessels.
But red blood cells with fetal hemoglobin don’t sickle. The newly-approved Casgevy gene therapy restarts fetal hemoglobin production by using CRISPR gene editing to dial down a gene called BCL11A.
“BCL11A represses fetal hemoglobin and also activates beta hemoglobin, which is affected by the sickle-cell mutation,” explains Stuart Orkin, MD, whose lab led the way in establishing BCL11A as a treatment target. “When you knock BCL11A down, you simultaneously increase fetal hemoglobin and repress sickle hemoglobin.”
Multiple discoveries made this treatment possible, the first dating back 75 years. Boston Children’s seminal contributions to sickle cell gene therapy reach back to the 1980s, leading to both Casgevy and a gene-transfer approach developed at Boston Children’s (now in Phase 2 clinical trials).
1948: Fetal hemoglobin doesn’t sickle
Brooklyn pediatrician Janet Watson notes that children with sickle cell disease had few sickled red blood cells as newborns. She attributes this to newborns’ greater concentration of fetal hemoglobin, which blocks the sickling process.
1949: Discovery of the sickle cell defect
Famed chemist Linus Pauling and associates show in the journal Science that oxygen-carrying hemoglobin has an altered chemical structure in people with sickle cell disease.
1956: Genetic change pinpointed in sickle hemoglobin
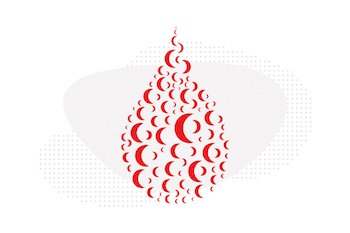
Reporting in Nature, Vernon Ingram at the University of Cambridge, U.K., identified the precise difference between sickle hemoglobin and normal hemoglobin: a single amino acid replacement at position 6 of the beta-globin protein.
1972-1990s: Fetal hemoglobin makes sickle cell milder
Studies of adults with a hereditary condition causing them to continue making fetal hemoglobin show that the persistence of fetal hemoglobin makes sickle cell disease milder.
1980s: The development of gene delivery vectors
The era of virus “vectors” that deliver genes into cells begins. Two teams — one led by David Williams, MD, at Boston Children’s Hospital and the other at the Hospital for Sick Children in Toronto — use retroviruses to transfer genes into blood stem cells. This step paves the way for future gene therapies.
1994: Fetal hemoglobin boosts life expectancy
A 23-center U.S. study led by Orah Platt, MD, at Boston Children’s finds that people with sickle cell disease live longer if they have higher levels of fetal hemoglobin.
1998: FDA approves hydroxyurea as a sickle cell drug
Building on early work done by Platt and David Nathan, MD, at Boston Children’s, and a subsequent clinical trial, the FDA approves hydroxyurea, a drug that indirectly increases fetal hemoglobin production and reduces the number and severity of sickle cell attacks. However, hydroxyurea only helps about half of all patients, and is not curative.
2008: A fetal hemoglobin silencer: BCL11A
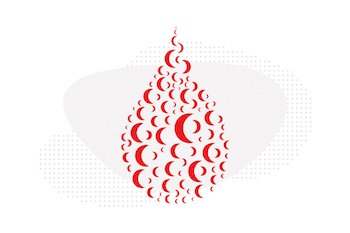
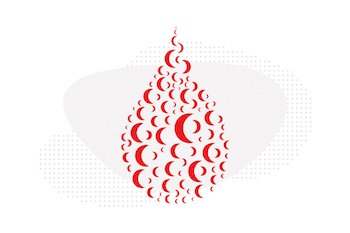
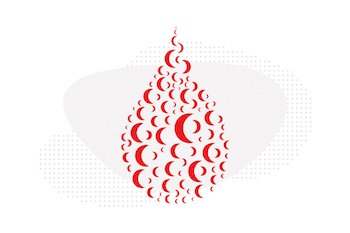
Two seminal studies launch a new approach to reactivating fetal hemoglobin production: gene therapy. First, an NIH-supported genome-wide association study led by Orkin and Joel Hirschhorn, MD, PhD, of Boston Children’s and the Broad Institute, suggests that variants of the gene BCL11A affect fetal hemoglobin production. Orkin and Vijay Sankaran, MD, PhD, at the Dana-Farber/Boston Children’s Cancer and Blood Disorders Center, then show that suppressing BCL11A restarts fetal hemoglobin production.
2011: Curing sickle cell in mice
In a key proof-of-principle, Orkin and colleagues correct sickle-cell disease in mice by inactivating BCL11A, allowing fetal hemoglobin to be made. These experiments showed that modulation of BCL11A alone is sufficient to correct the disease.
2013-2015: A safer way to enhance fetal hemoglobin
Scientists led by Orkin and Daniel Bauer, MD, PhD, at Dana-Farber/Boston Children’s, find a region of DNA within the BCL11A gene, a so-called “enhancer” region, that is required for production of BCL11A, but only in red blood cells, providing a safer target for inducing fetal hemoglobin production. Using newly discovered CRISPR editing, they identify a discrete site within the enhancer that confers much of its total activity and can be edited by CRISPR with high efficiency, leading to robust fetal hemoglobin expression. The work sets the stage for future gene editing therapies for sickle cell disease.
2016: A first gene therapy vector targeting BCL11A
Led by David Williams, MD, Dana-Farber/Boston Children’s researchers create a precision-engineered gene therapy vector that down-regulates BCL11A only in precursors of red blood cells. Its core is a short hairpin RNA embedded in a cellular microRNA (called an shmiR) that inactivates the BCL11A gene only in red blood cell precursors, delivered by a lentivirus engineered to be safe in humans. This vector achieved fetal hemoglobin levels well above those needed to prevent sickling in red blood cells from mice and four patients with sickle cell disease.
2017: First sickle cell gene transfer trial opens
Using this lentiviral vector, a Phase 1, open-label trial begins enrolling patients 18 and older with severe sickle cell disease, led by Williams and Erica Esrick, MD. The gene therapy is delivered through a stem cell (bone marrow) transplant.
2018: First sickle cell gene editing trial opens
The treatment now known as Casgevy enters a clinical trial sponsored by CRISPR Therapeutics and Vertex Pharmaceuticals. Based on the work by Orkin, Bauer, and colleagues, it uses CRISPR-Cas 9 to edit the BCL11A enhancer at a particularly vulnerable site.
January 2021: First clinical trial results
Two separate clinical trials report positive results for both gene therapy approaches developed at Boston Children’s. The first trial, published in The New England Journal of Medicine, was based on the work by Orkin and Bauer. It tested CRISPR gene editing in two patients — one with sickle cell disease and one with beta thalassemia.
In the same issue of the journal, Esrick and Williams report the results of gene therapy to silence BCL11A in six patients with sickle cell disease, using the lentiviral vector developed by Williams and colleagues. After treatment, patients show robust levels of fetal hemoglobin production and reduced (or even absent) symptoms.
July 2022: GRASP trial opens
A national, multi-center Phase 2 trial, sponsored by Williams, continues to test gene therapy with the lentiviral vector. Adolescents as young as 13 are eligible to enroll in the trial, known as the Gene Transfer Study Inducing Fetal Hemoglobin in Sickle Cell Disease trial (GRASP).
November/December 2023: First sickle cell gene therapy approvals
Sickle cell gene therapy using CRISPR editing, based on the technology developed by Orkin and Bauer, is approved in the U.K. on November 16 and by the U.S. FDA on December 8. Manufactured as Casgevy by Vertex and CRISPR Therapeutics, it is one of two sickle cell gene therapies approved by the FDA for patients 12 and older. (The other therapy, known as Lyfgenia, uses a different approach not based on fetal hemoglobin.) Casgevy is also the world’s first CRISPR-based gene therapy for any disease to gain regulatory approval.
Now available to patients with sickle cell disease
Boston Children’s Gene Therapy Program will soon be offering Casgevy to patients age 12 and older. Alternatively, children as young as 13 can enroll in the GRASP trial to receive gene therapy with the lentiviral vector. All three gene therapies — Casgevy, Lyfgenia, and the lentiviral gene therapy — have only been in use for a relatively short time, so hematologists will be watching carefully to see how lasting the effects are and whether there are any long-term side effects.
Ultimately, Orkin hopes to develop a drug that would reduce BCL11A and raise fetal hemoglobin at a much lower cost. “We need a small molecule treatment that mimics what genetics can do to make treatment available for a much wider population,” Orkin says. “We’re working on this in the lab right now.”
Visit the Gene Therapy Program to learn more about gene therapy treatments for sickle cell disease and other conditions.
Related Posts :
-
A first for CRISPR: Cutting genes in blood stem cells
CRISPR — a gene editing technology that lets researchers make precise mutations, deletions and even replacements in genomic DNA — is all ...
-
After decades of evolution, gene therapy arrives
As early as the 1960s, scientists speculated that DNA sequences could be introduced into patients’ cells to cure genetic disorders. ...
-
Manny: Hoping new research helps others with sickle cell disease
Emmanuel “Manny” Johnson, Jr., shares many loves with his little brother, Aiden — from basketball to video games. One thing he ...
-
Sickle cell disease, gene therapy, and blood cancers: Mysteries remain
Gene therapy trials for sickle cell disease have been showing great promise, even offering hope of a cure. But in ...