After decades of evolution, gene therapy arrives
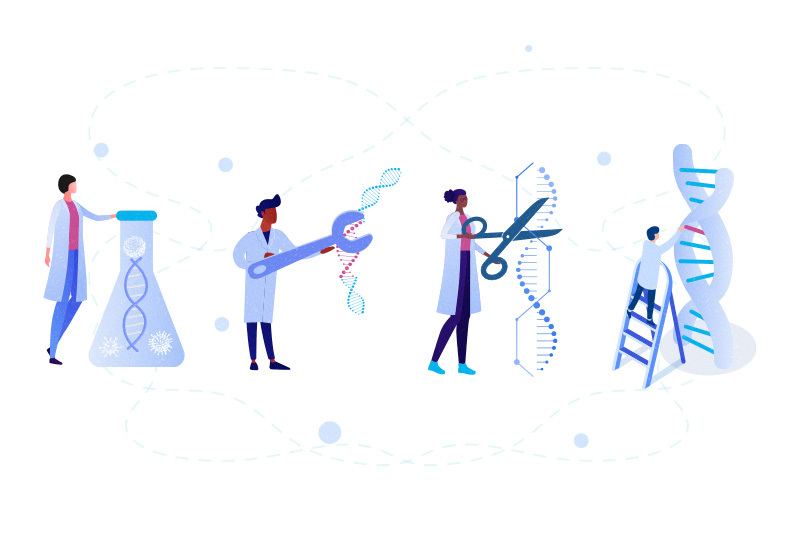
As early as the 1960s, scientists speculated that DNA sequences could be introduced into patients’ cells to cure genetic disorders. In the early 1980s, David Williams, MD, and David Nathan, MD, at Boston Children’s Hospital published the first paper showing one could use a virus to insert genes into blood-forming stem cells. In 2003, the Human Genome Project wrapped up, giving us a complete blueprint of our DNA. In the past decade, gene therapy has become a reality for multiple diseases, especially those caused by mutations in a single gene.
Gene therapy falls into two main categories. Ex vivo gene therapy removes cells from the patient, introduces new genetic material, packaged in a delivery vehicle called a vector, then returns the cells to the patient. Boston Children’s is using this method for such disorders as sickle cell disease, adrenoleukodystrophy, chronic granulomatous disease and others. In vivo gene therapy involves direct IV infusion of the vector into the bloodstream or injection into a target organ like the eye. Boston Children’s uses in vivo gene therapy for several disorders, including hemophilia and ornithine transcarbamylase deficiency.
After a rocky start, gene therapy is on fire, drawing keen interest from the biopharmaceutical industry. And it’s still evolving and improving.
Gene therapy 1.0: First introduction of corrected genes
In 1990, 4-year-old Ashanthi de Silva became the first gene therapy success story. She was born with a severe combined immunodeficiency (SCID) due to lack of the enzyme adenosine deaminase (ADA). Without ADA, her T cells died off, leaving her unable to fight infections. Injections of a synthetic ADA enzyme helped, but only temporarily.
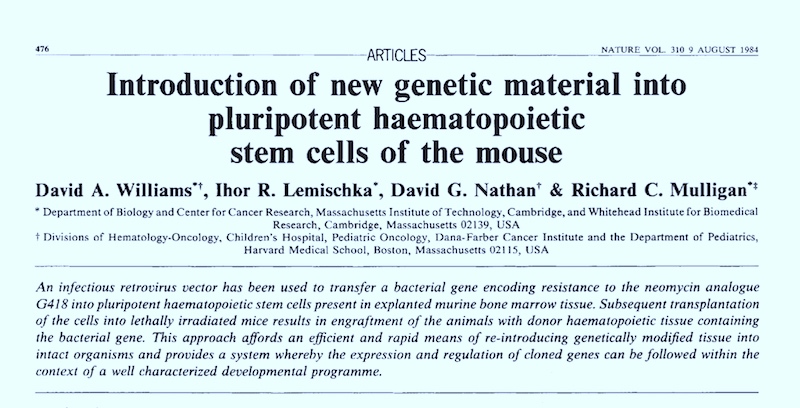
Doctors decided to deliver a healthy ADA gene into her blood cells, using a disabled virus that cannot spread in the body. Their success spurred more trials in the 1990s for the same form of SCID. Now in her 30s, de Silva is active in the rare disease community.
European researchers in the 1990s focused on SCID-X1, another form of SCID linked to the X chromosome. They reported the first cures in 2000, but within several years, five of the 20 treated children developed cancer. The viral vector that delivered the gene to their T cells had also activated an oncogene, triggering leukemia.
The U.S. saw another early setback: the 1999 death of 18-year-old Jesse Gelsinger, after receiving gene therapy for a rare metabolic disorder. In his case, the viral vector caused a fatal immune response.
Gene therapy came to a halt.
Gene therapy 2.0: Improved viral vectors
In the early 2010s, gene therapy experienced a renaissance. Scientists developed better viral vectors to deliver genetic therapies. They added regulatory elements called promoters and enhancers to direct the gene’s activity. These elements specified where and when the gene should turn on, and at what level. Investigators at Boston Children’s, in a global collaborative effort, led work that addressed the problem of leukemia, allowing gene therapy to resume for SCID-X1.
A REBIRTH IN BOSTON: GENE THERAPY TURNS 10
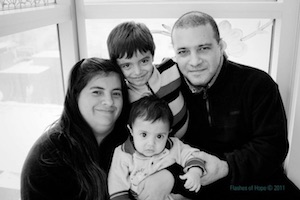
Born in 2010 with X-linked severe combined immunodeficiency (SCID-X1), Agustín spent the first few months of his life in isolation. He became the first patient to receive gene therapy at Boston Children’s and today is an active fifth-grade soccer and tennis player.
The new, modified vectors can more precisely target expression of genes in specific cell types, don’t go astray in the body, and don’t trigger the immune system. Some deliver genes meant to work for a short while and then inactivate themselves. Others carry genes that remain active long-term and pass to daughter cells as the cells divide. Popular viruses for gene therapy include adenoviruses, adeno-associated virus, and lentiviruses.
An example of an improved vector is the lentivirus vector used for sickle cell gene therapy at Boston Children’s. The vector silences a gene called BCL11A, leading to production of fetal hemoglobin that is not affected by the sickle cell mutation. It was precision engineered to silence the gene only in precursors of red blood cells, a tweak that enabled the treated blood stem cells to live long-term in patients’ bone marrow. Williams led the vector’s development, based on seminal research by Vijay Sankaran, MD, PhD, and Stuart Orkin, MD, in the Hematology/Oncology Program at Boston Children’s.
Gene therapy 3.0: Gene editing and base editing
Traditional gene therapy uses viruses to carry healthy genes into cells, compensating for a faulty or missing gene. But the past decade has seen an explosion of other methods for delivering or fixing genes.
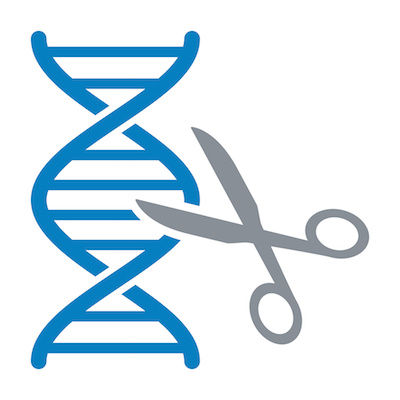
Gene editing uses various molecular tools that precisely target problematic genes and create a cut or break in their DNA. It can knock out a faulty gene, insert a new DNA sequence, or both in a “cut and paste” operation. The best-known gene editing systems are CRISPR/Cas 9, zinc-finger nucleases (ZFNs), and transcription activator-like effector nucleases (TALENs). The next generation of gene therapy for sickle cell disease is utilizing CRISPR to edit the BCL11A gene, based on work by Dan Bauer, MD, PhD, at Boston Children’s, then in Orkin’s laboratory.
Base editing is even more fine-tuned. It leverages the targeting ability of CRISPR, but relies on enzymes to chemically change one “letter” of a gene’s code at a time — changing, say, C to T or A to G. These small changes can correct a “spelling error” mutation, silence a disease-causing gene, or help activate a specific gene. Unlike gene editing, base editing hasn’t yet been tested in clinical trials, but it offers the promise of more precision, efficiency, and safety. Boston Children’s has several base editing projects on deck.
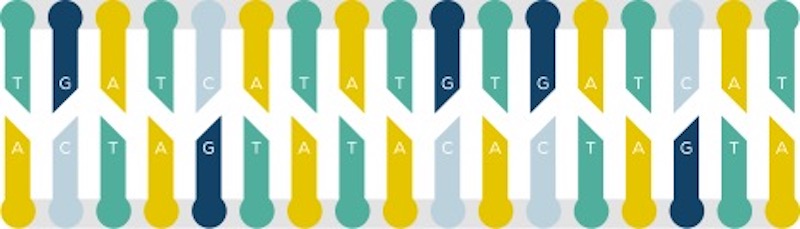
Other genetic therapies
Other new approaches blur the line between gene therapy and drug treatment. For example, antisense oligonucleotides (ASOs) are drugs made up of short, synthetic pieces of DNA or RNA that target the messenger RNA made by the faulty gene. They prevent the gene from being translated into a “bad” protein or, in some cases, trick the cell’s machinery into making a “good” protein. Researchers can even customize ASOs to single patients. Tim Yu, MD, PhD, in the Division of Genetics and Genomics at Boston Children’s, has developed this approach to treat several very rare genetic conditions.
Another approach, RNA interference, uses small RNAs to “silence” a targeted gene by neutralizing the gene’s mRNA. (The lentivirus described above uses RNA interference to silence the BCL11A gene.)
Even the messenger RNAs used for some COVID-19 vaccines represent a form of gene therapy. The mRNAs introduce genetic code that cells then use to make the coronavirus spike protein, encouraging people to develop antibodies to the virus.
Today, ClinicalTrials.gov lists nearly 400 active gene therapy studies all over the world, and more than a dozen gene therapy drugs are on the market. At Boston Children’s, the Gene Therapy Program has more than 20 human trials completed or underway, with more in the pipeline. While gene therapies are currently expensive, it’s expected that prices will come down over time. And as a one-time treatment, gene therapy promises to save money in the long run by preventing a lifetime of illness — a true revolution in medicine.
Learn more about the Gene Therapy Program at Boston Children’s Hospital
Related Posts :
-
New research sheds light on the genetic roots of amblyopia
For decades, amblyopia has been considered a disorder primarily caused by abnormal visual experiences early in life. But new research ...
-
Parsing the promise of inosine for neurogenic bladder
Spinal cord damage — whether from traumatic injury or conditions such as spina bifida — can have a profound impact on bladder ...
-
Thanks to Carter and his family, people are talking about spastic paraplegia
Nine-year-old Carter may be the most devoted — and popular — sports fan in his Connecticut town. “He loves all sports,” ...
-
Unveiling the hidden impact of moyamoya disease: Brain injury without symptoms
Moyamoya disease — a rare, progressive condition that narrows the brain’s blood vessels — leads to an increased risk of stroke ...