Gene therapy’s future may be all about the bases
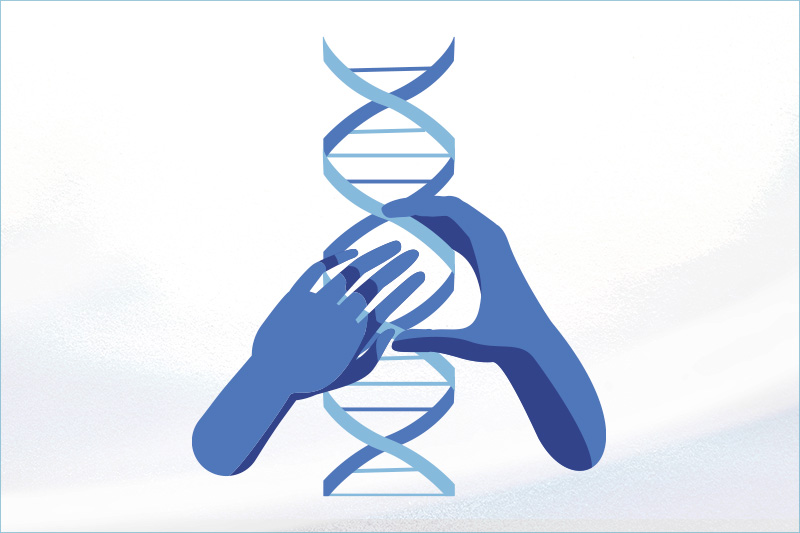
Gene therapy offers the possibility of a cure for many genetic disorders, especially those involving a single gene. The first kind of gene therapy used a virus to carry a corrected copy of the gene into people’s cells. When the early viral vectors used in the 1990s were found to have off-target effects, sometimes even causing cancer, investigators refined them to be safer.
David Williams, MD, who founded Boston Children’s Hospital’s gene therapy program in 2010, was instrumental in this effort. He had helped develop viral vector technology as a hematology/oncology fellow in the early 1980s. Today, most of the hospital’s current gene therapy trials still involve viral vectors.
A newer method, gene editing, uses enzymes that cut DNA to delete, insert, or correct genes. Gene editing is now in multiple clinical trials, including a beta-thalassemia trial at Boston Children’s. But there are still theoretical safety concerns.
“Any time you cut DNA, you worry about creating a mutation that you didn’t intend,” says Williams, now Boston Children’s chief scientific officer.
Williams has his sights set on the future. And that future will involve base editing — which might prove to be the safest, most precise form of gene therapy yet.
A strategic alliance
To explore base editing, the hospital has just formed a strategic alliance with Beam Therapeutics, a Cambridge-based biotechnology company that is pioneering base editing methods. Under the three-year agreement, Beam will sponsor multiple research programs at Boston Children’s to facilitate development of disease-specific therapies using Beam’s base-editing technologies.
“We benefit from their scientific innovation, and they benefit from our significant background in doing gene therapy trials, including regulatory experience, as well as access to our top scientists and clinical programs,” says Williams. “We see this as another step in the continued development of our gene therapy program.”
Gene therapy 3.0
Base editing is like spell correction for the genome. It creates precise changes in the genetic code, one “letter” at a time. The letters are bases, the smallest building blocks of our genome. There are four: adenine (A), cytosine (C), guanine (G), and thymine (T).
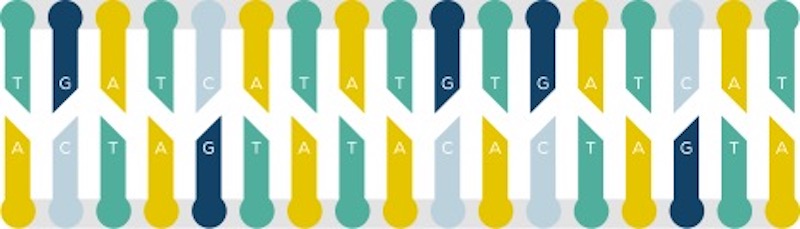
Unlike gene editing strategies such as CRISPR, which create a cut or break in the double strand of DNA, base editing uses separate components for targeting and editing. A CRISPR protein, modified not to cause double-stranded breaks, targets a specific location in the genome. A base editing enzyme, such as a deaminase, then carries out the desired chemical modification. Beam’s base editors chemically transform one base into another — changing C to T, or A to G. These small changes can correct a point mutation, silence a disease-causing gene, or help activate a specific gene.
Traditional gene therapy uses a virus to carry healthy copies of a gene into cells, compensating for a faulty or missing gene in the genome.
CRISPR enables precise targeting of DNA sequences, making a double-stranded break in the DNA at the targeted location.
Base editing leverages the targeting ability of CRISPR, but relies on targeted enzymes to chemically change one base to another, correcting a single “letter” of a gene’s code.
Williams thinks base editing offers a number of potential advances over existing gene editing methods: precision, predictable editing outcomes, high editing efficiency, and greater safety. But he cautions that it’s an emerging approach that hasn’t yet been tested in clinical trials.
“It’s still very early,” he says. “Whether base editing approaches will be as successful as viral vector gene therapy is what we hope to find out through our collaboration. One of the keys to success is how efficient this approach will be in large, clinical-scale projects.”
Base editing projects on deck
The collaboration will start with blood disorders in which blood cells can be removed from the body, treated, and returned to the patient — so-called “ex vivo” gene therapy.
Preclinical studies from Beam have successfully reproduced single-base changes observed in people with hereditary persistence of fetal hemoglobin. This resulted in long-term engraftment and therapeutically relevant increases in gamma globin protein expression in vivo.
“The exciting plan is to explore this technology to change single-base-pair mutations causing disease, such as those we see in sickle cell disease, to non-disease sequences,” says Williams.
Down the road, Beam may sponsor additional research projects where the company’s and hospital’s interests align.
“As the technology evolves, we’re hoping to apply gene therapy to a broader spectrum of diseases than we have in the past, and to do that in a way that’s safer,” says Williams.
Learn more about Boston Children’s Gene Therapy Program.
Related Posts :
-
New research sheds light on the genetic roots of amblyopia
For decades, amblyopia has been considered a disorder primarily caused by abnormal visual experiences early in life. But new research ...
-
Thanks to Carter and his family, people are talking about spastic paraplegia
Nine-year-old Carter may be the most devoted — and popular — sports fan in his Connecticut town. “He loves all sports,” ...
-
“A setback for a comeback”: Brody perseveres with Paget-Schroetter Syndrome
Baseball has been part of Brody Walsh’s story from the very start. Now 19 and a college sophomore, Brody pitches ...
-
Tough cookie: Steroid therapy helps Alessandra thrive with Diamond-Blackfan anemia
Two-year-old Alessandra is many things. She’s sweet, happy, curious, and, according to her parents, Ralph and Irma, a budding ...