Tissue models and a gene therapy for a deadly heart arrhythmia
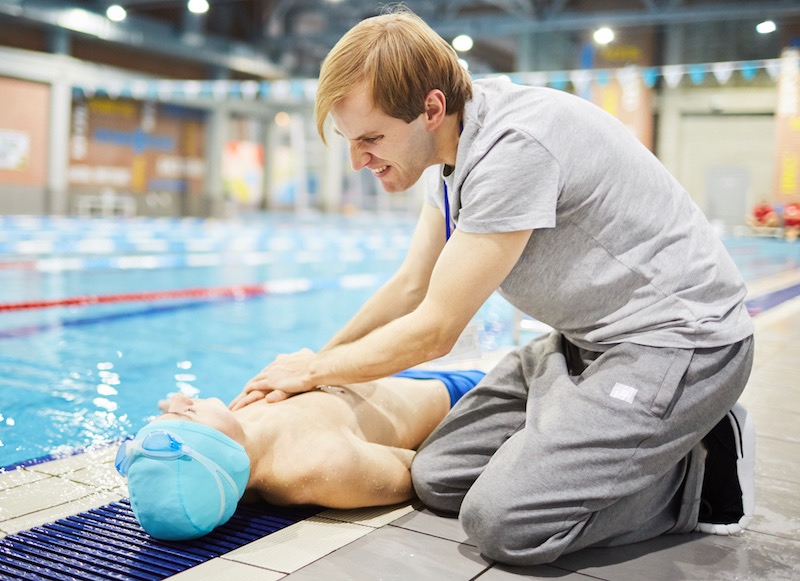
Catecholaminergic polymorphic ventricular tachycardia (CPVT) is a leading cause of sudden death from cardiac arrest in children and young adults. Marked by racing, irregular heartbeats, the inherited arrhythmia is typically silent until a child suddenly collapses and faints, at an average age of 12. The trigger is an adrenaline surge, caused by exercise or emotional stress.
In a pair of new studies in the July 30th print issue of Circulation, researchers at Boston Children’s Hospital report creating the first human tissue model of CPVT, replicating two patients’ abnormal heart rhythms, and then suppressing the arrhythmia with gene therapy in a mouse model. The work opens the possibility of genetically correcting CPVT and other inherited arrhythmias, and perhaps more common arrhythmias like atrial fibrillation.
“Our hope is to give gene therapy in a single dose that would work indefinitely,” says Vassilios Bezzerides, MD, PhD, an attending cardiologist in Boston Children’s Inherited Cardiac Arrhythmias Program, who was involved in both studies. “Our work provides proof-of-concept for a translatable gene therapy strategy.”
Improving treatment options for CPVT
Current treatment for CPVT consists of drugs such as beta-blockers and flecainide, surgery to disconnect nerves innervating the left side of the heart, and an implanted cardioverter-defibrillator (ICD). But these approaches often don’t work, and ICDs can actually be dangerous in CPVT, inducing a life-threatening “electrical storm.” Often, children’s main option is simply avoiding physical and emotional exertion.
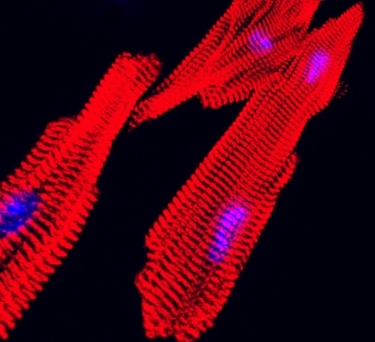
“Treatments for CPVT are currently pretty inadequate,” says Bezzerides. “Twenty-five to 30 percent of patients will have recurrent life-threatening arrhythmias despite treatment.”
Seeking new approaches, William T. Pu, MD, of Boston Children’s Hospital and Kevin Kit Parker, PhD, of Boston Children’s and Harvard’s School of Engineering, Arts, and Sciences (SEAS), co-senior authors on the first study, more detailed understanding of how CPVT works. As described online today in Circulation, they set out to build tissue models that faithfully replicated CPVT in a dish, using blood samples from two patients.
Building arrhythmic tissue
Both patients had a mutation in RYR2, the gene linked to most cases of CPVT. RYR2 encodes a channel that enables cells to release calcium — the first step in initiating a heart contraction. The scientists first reprogrammed their blood cells to become induced pluripotent stem (iPS) cells, capable of making virtually all cell types. From these, they made cardiomyocytes (heart muscle cells) carrying the CPVT mutations, and seeded these cells onto an engineered surface.
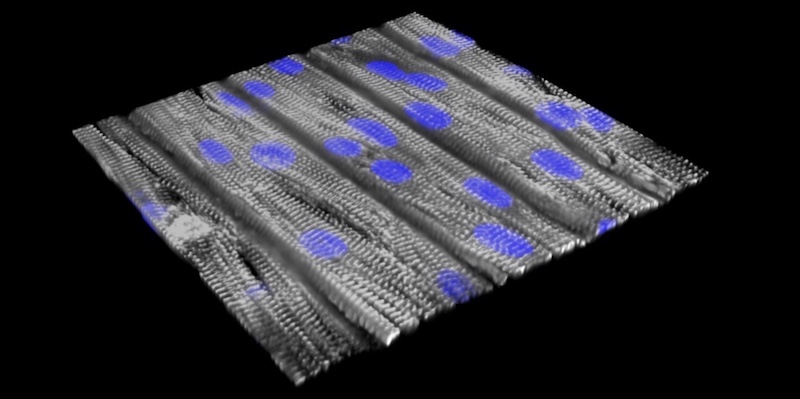
“The cells lined up in a specific direction similar to how heart muscle is organized,” says Pu, who directs Basic and Translational Cardiovascular Research at Boston Children’s. “The cells have very abnormal beating individually, but after assembly into tissue, they beat together, better modeling the actual disease. That’s why tissue-level models are important.”
Exercise test in a dish
The team next created an “exercise test in a dish.” Using a so-called optogenetic system, they applied blue light to one end of the tissue, activating the cells and creating an impulse that moved along the sheet of cells to produce a contraction. To simulate exercise, they added the drug isoproterenol (similar to the stress hormone adrenaline) and applied infrared light to initiate faster heartbeats. (Video: Sung Jin Park, Parker Laboratory and Donghui Zhang, Pu Laboratory.)
This testing helped reveal CPVT’s underlying mechanisms. When healthy heart tissue underwent the exercise test, calcium moved through the tissue in even waves. But in tissue models made from the patients with CPVT, the calcium waves moved at varying speeds, and in some parts of the tissue not at all. This resulted in an abnormal circular motion known as re-entry, much like what happens in real life.
“When we paced the cells faster, the CPVT tissue sustained re-entrant arrhythmias, whereas normal tissue could handle it fine,” says Pu.
Finding a target with CRISPR
Why does stress make CPVT patients vulnerable to life-threatening arrhythmias? To find out, Pu, Parker, and colleagues identified signaling molecules that are activated by adrenaline. They then used drugs and CRISPR/Cas9 genome editing to selectively inhibit or modify them.
Through this strategy, they found that an enzyme called CaM kinase (CaMKII) chemically modifies RYR2, triggering cardiomyocytes to release more calcium. In healthy heart tissue, this causes no problem, but in CPVT, this modification combines with the inherited RYR2 mutation to produce excessive calcium levels in cells, precipitating arrhythmias.
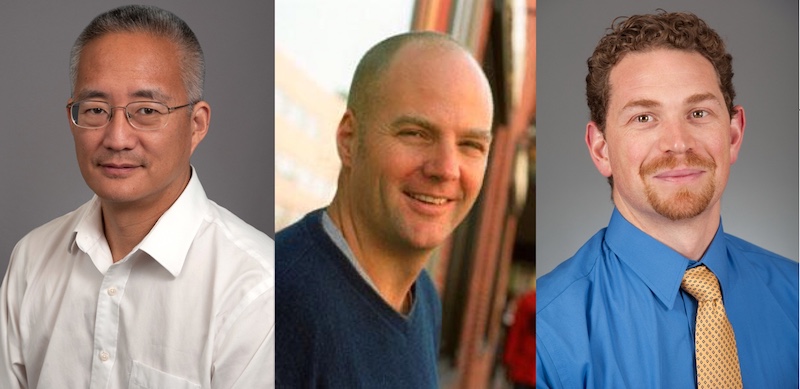
“Nature designed CaMKII as part of the fight-or-flight response,” explains Pu. “When you get excited, you release more calcium so the heart can beat faster. But when RYR2 is mutated, the channel is leaky, so the cell releases way too much calcium, which causes arrhythmia.”
When the researchers blocked the CaMKII modification, they eliminated the arrhythmias in the CPVT tissue model. They got the same effect when they blocked CaMKII itself with a potent and selective inhibitor, a peptide called AIP.
“The coupling of iPS technology and ‘organs on chips’ offers new opportunities for studies in precision medicine,” says Parker. “By replicating the patient’s disease in vitro, we can test candidate therapies and measure safety and efficacy, so that the right patients get tested with the right drug.”
Inhibiting CPVT with gene therapy
In a separate study, published online by Circulation on June 3, a team led by Bezzerides tested a gene therapy approach to inhibiting CaMKII in a mouse model of CPVT. Because CaMKII acts on many tissues beside the heart — in fact, it’s needed by the brain to form memories — the team wanted to be able to inhibit the enzyme only in the heart.
They engineered a special virus which, injected into mice, selectively travelled to the heart and delivered AIP. Testing found AIP in about 50 percent of heart cells, enough to suppress arrhythmias, but not in other tissues, including the brain.
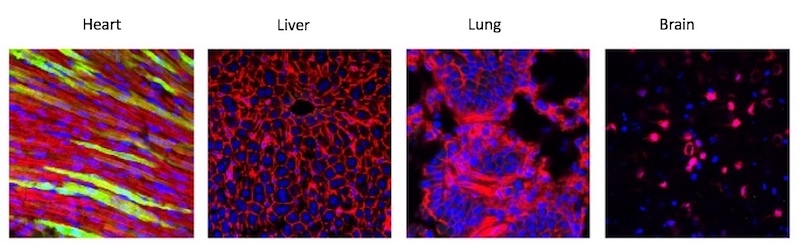
Pu, senior author on the paper, plans to refine the gene therapy strategy together with Bezzerides and test it in a large animal model and eventually in patients with CPVT. Clinical testing would likely be carried out in collaboration with other medical centers.
A general approach to heart disease?
Bezzerides and Pu believe gene therapy could be effective for patients with CPVT caused by a variety of RYR2 mutations (more than 160 mutations have been reported). And they believe their overall strategy of inhibiting CaMKII in the heart could help treat more common causes of heart disease.
“CaMKII is not needed for normal heart function, but it becomes activated in many forms of heart disease,” says Pu. “In mouse models of many forms of heart disease, such as ischemic cardiomyopathy, atrial fibrillation, or hypertrophic cardiomyopathy, chronic CaMKII activation is detrimental. It is possible that our gene therapy approach to CaMKII inhibition could improve outcomes in these other types of heart disease.”
Vassilios Bezzerides was first author on the gene therapy study, which was funded by the National Institutes of Health, the American Heart Association, Boston Children’s Heart Center, the Mannion and Roberts families, and the Sarnoff Cardiovascular Research Foundation.
Sung-Jin Park of Harvard SEAS and Donghui Zhang of Boston Children’s were co-first authors on the CPVT tissue study. Funders included the National Institutes of Health, the Boston Children’s Translational Investigator Service, the Boston Children’s Heart Center, the Mannion and Roberts families, SEAS, the Wyss Institute, the National Science Foundation and the American Heart Foundation. See the papers for a complete list co-authors and funders.
Related Posts :
-
Lucas receives gene therapy for DMD and finds his super muscles
Lucas Toro has a lot in common with Gekko, the cartoon character from PJ Masks. They’re both “little guys” ...
-
Mending injured hearts: Lessons from newborns?
When the heart is injured, as in a myocardial infarction, the damaged heart muscle cannot regenerate — instead, scar tissue forms. ...
-
In the genetics of congenital heart disease, noncoding DNA fills in some blanks
Researchers have been chipping away at the genetic causes of congenital heart disease (CHD) for a couple of decades. About 45 ...
-
The journey to a treatment for hereditary spastic paraplegia
In 2016, Darius Ebrahimi-Fakhari, MD, PhD, a neurology fellow at Boston Children’s Hospital, met two little girls with spasticity and ...