New stem cell pipeline makes more lifelike cell products
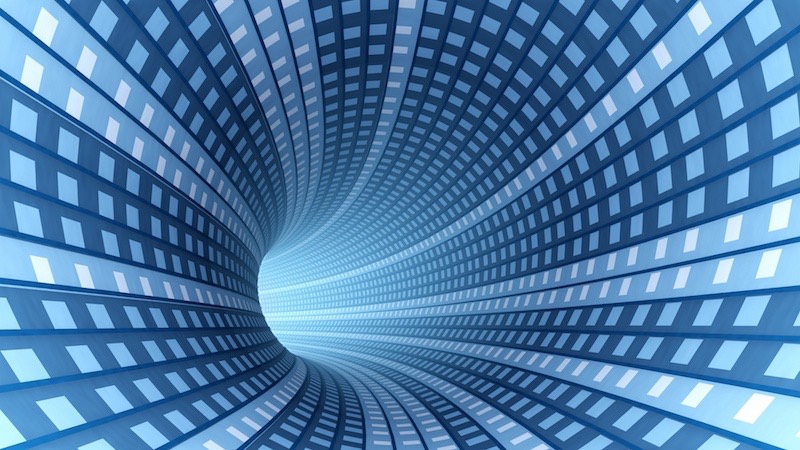
Researchers in many fields of science and medicine engineer different types of human cells for drug testing and other purposes. They often begin with stem cells — unspecialized cells that have the potential to form many different types of tissue. By turning on certain genes, the scientists attempt to coax the stem cells into becoming a particular type (heart, liver, skin, etc.), mimicking the natural process of cell differentiation that occurs during embryonic development. But they’re left with the question: How close are my engineered cells to the real thing?
Five years ago, scientists at Boston Children’s Hospital and other institutions designed a computer algorithm called CellNet to help answer that question. Scientists anywhere in the world could take certain measurements of their cells and upload that data via the internet. CellNet would then give them a numerical score indicating how similar their cells were to bona fide human cells of that type — and suggest steps they could take to make their cells more like their natural counterparts.
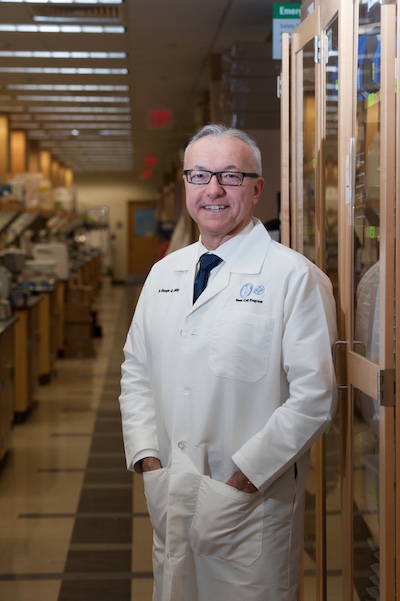
Now, in a paper published July 1 in Nature Biotechnology, some of the investigators behind CellNet unveil a “systems biology pipeline.” They show how readily available data on protein interaction networks and gene expression can augment the CellNet algorithm to capture the complexity of differentiation and thereby create more lifelike cells.
“Stem cell engineering requires the integration of powerful datasets to achieve the most refined cellular products that most closely mimic their native counterparts,” says George Daley, Dean of Harvard Medical School, a principal investigator in Boston Children’s Stem Cell Research program, and a co-senior author of the paper. “Our systems biology pipeline will enhance the generation of cell products.”
Test case: blood cells
In 2014, Melissa Kinney, PhD, then a postdoc in Daley’s lab, set out to explore whether CellNet could distinguish between the many intermediate stages that stem cells go through as they differentiate. As a test case, she chose blood cells, because they’re well studied and have many types and subtly different stages of development.
To track this down, we ended up getting off the computer and going into the lab.
Kinney, who is lead author on the new paper, found that CellNet could take her only so far. The algorithm could distinguish hematopoietic stem cells — the immature progenitors of all blood cells, found in bone marrow — from the mature red blood cells they ultimately become. But it could not parse out the intermediate stages. So Kinney turned to the systems biology expertise of the laboratory of Doug Lauffenburger, PhD, head of the biological engineering department at the Massachusetts Institute of Technology.
When switching on genes isn’t enough
In the last few years, scientists have learned that simply switching on certain genes is not enough to turn a stem cell into a particular type of cell. During the natural process of development in the body, each cell interacts with the other cells around it. Those contacts result in molecular messages being sent from the surface of the cell via specific signaling pathways to the nucleus, where the genes reside. And proteins called transcription factors are involved in turning multiple genes on and off. The Lauffenburger lab helped Kinney understand how these and other factors form a complex regulatory network that determines a cell’s fate.
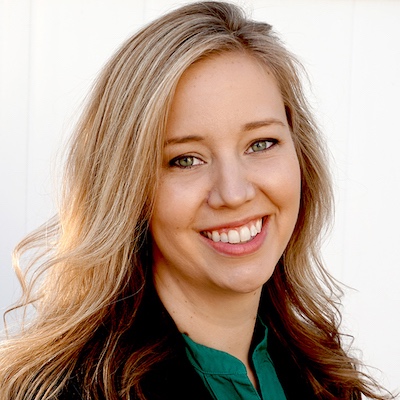
To track the stages developing blood cells go through, Kinney and her colleagues fed data from publicly available sources into a computational algorithm to find the set of genes that distinguish stem cells from differentiated red blood cells. Then they went through two more stages of calculations to determine how the genes work together during the intermediate stages of differentiation.
“What you flow into the computational pipeline is data, and what you get out is progressively more specific information about how to distinguish differentiating cells,” she explains.
Kinney was then able to use that information to tweak the blood cells in her petri dishes, adding specific growth factors or small molecules to nudge the cells into being more like the natural cells they’re meant to emulate.
A surprising discovery
In exploring the multiple stages of blood cell development, Kinney made a surprising discovery. Her computational analysis kept turning up signs of a protein called ErbB. That was counterintuitive, she says, because ErbB proteins have been associated with cancer, in which cells revert to a less mature state and grow unchecked — “almost the opposite of differentiation,” she says. “And so, to track this down, we ended up getting off the computer and going into the lab to try to figure out what was happening.”
By using drugs to perturb the ErbB pathway in mouse, zebrafish, and human cells, Kinney and her colleagues were able to confirm that a relatively unknown family member called ErbB4 is required for blood generation. “We were able to track this hypothesis all the way from the computer into model organisms, which I think was one of the most exciting parts of this paper,” she says.
Kinney, now an assistant professor of biomedical engineering at the University of Wisconsin-Madison, hopes the analytical methods used in the Nature Biotechnology paper will offer other scientists a roadmap for studying many different cell types and more precisely defining the stages they go through as they differentiate.
Clinical implications
The work also has a number of clinical implications, Kinney says. For example, uncovering the importance of ErbB4 in blood cell development may shed light on why anemia is such a common complication of cancer treatments that target the ErbB pathway. And the ability to create human cells of many types that are more like their natural counterparts may improve cell replacement therapies and accelerate drug design.
Today, Kinney says, potential drugs are often tested on engineered cells that bear little resemblance to those in the human body. Many times, pharmaceutical screens show promising results, only to fail in clinical trials.
“The question is: Can we create cells that are more faithful to the human body in order to better predict which drugs will actually work? And can we use the cells themselves as a ‘living drug’ to replace defective cells?”
Lauffenburger and Daley were co-senior authors on the paper. For a complete list of co-authors, see Nature Biotechnology. The work is supported by the National Institutes of Health, the National Science Foundation, the Leukemia and Lymphoma Society, the Howard Hughes Medical Institute, and the Manton Center for Orphan Disease Research.
Related Posts :
-
A small act of kindness: Blood donations get Sadie off the sidelines after her aplastic anemia diagnosis
In March of 2024, Sadie’s life was interrupted. A busy high school senior with classes to attend, soccer matches to ...
-
A universal gene therapy for Diamond-Blackfan anemia is poised for clinical testing
Diamond-Blackfan anemia (DBA), first described at Boston Children’s Hospital in 1938, is a rare blood disorder in which the bone ...
-
Curbing blood cancers by teaching immune cells to kill mutant stem cells
Blood stem cells, which give rise to all of our blood cell types, undergo a quality assurance process after they’...
-
Mitochondrial transfer restores heart muscle — but how?
Transferring mitochondria from a patient’s healthy skeletal muscle to damaged, ischemic heart tissue has been shown to restore heart ...