Discoveries promise new strides for spinal cord injury patients
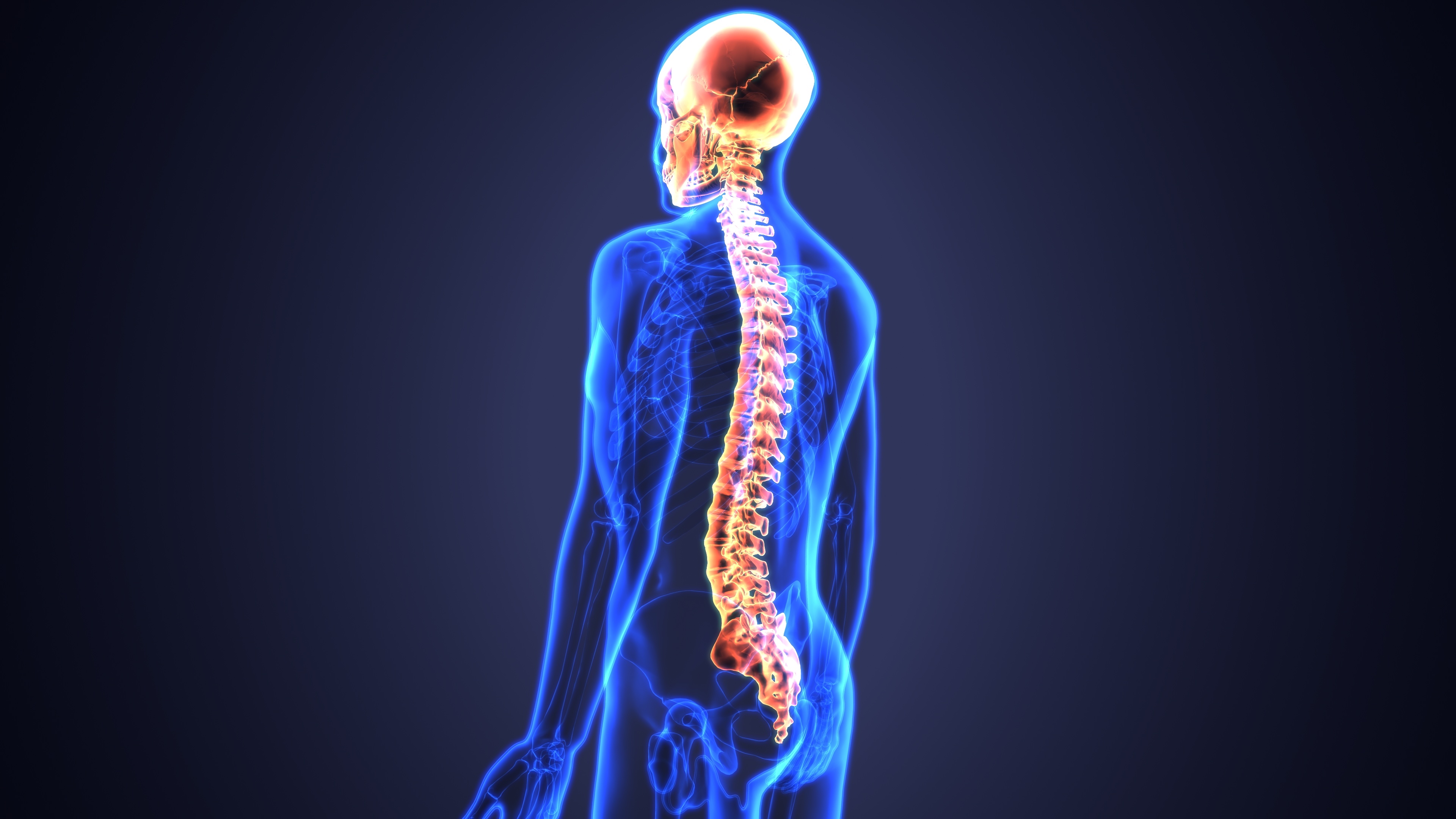
When neurobiologist Clifford Woolf, MB, BCh, PhD, began investigating potential treatments for spinal cord injury more than 30 years ago at University College London, he had mixed feelings about accepting funding for the research. “To be honest, the prospect that something could realistically make an impact on spinal cord injury in my lifetime seemed like science fiction,” he says.
Woolf, now the director of the F.M. Kirby Neurobiology Center at Boston Children’s Hospital, takes a decidedly more upbeat view today. Thanks to recent discoveries made at Boston Children’s and at other laboratories around the world, he believes the field is poised to develop treatments that significantly improve the lives of patients who up to now have endured lifelong paralysis and other medical problems.
“As a community, we’ve made more progress in the last ten years than we did in the previous 100 years,” says Kirby researcher Zhigang He, PhD, BM.
Progress to the point that He is now in discussions about beginning a clinical trial of an experimental treatment, discovered only last year, that enabled spinal cord-injured mice to walk again. “We’re very excited about it,” He says. “This is something we want to push as hard as possible.”
Conduit in the body’s communication system
The spinal cord is the major trunk line in the body’s intricate internal communication system. Running from the base of the brain to the tailbone, it carries signals from the brain out to the body’s limbs and organs, controlling our every action. In the other direction, it carries signals from the body back to the brain that allow us to see, hear, smell, taste, and feel.
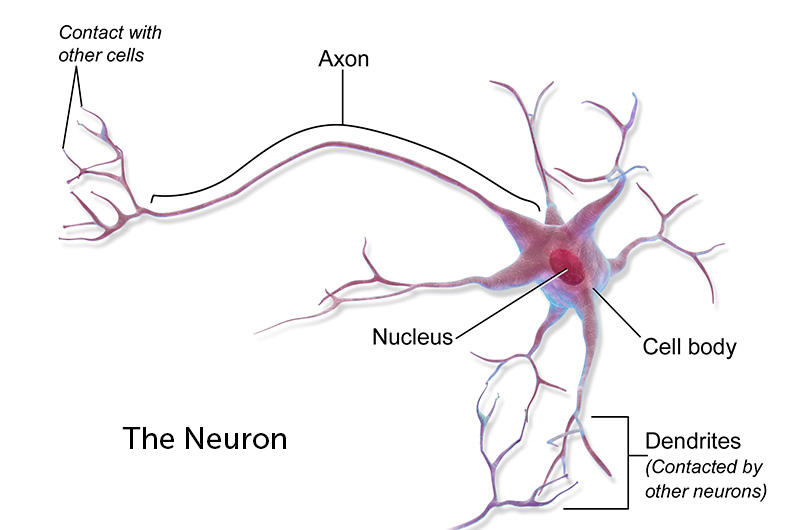
Passing all these signals along are neurons, nerve cells that act like radio relay stations. Extending from the cell body of each neuron are multiple, branching projections called dendrites — the “receivers” that take in impulses from surrounding nerve cells. Each neuron also has a “transmitter”— a single long tendril called the axon, which sends signals to other parts of the nervous system and the rest of the body. The longest axon extends from the base of the spinal cord to the big toe, a distance of about three feet.
The reason spinal cord injuries are so devastating is that the central nervous system, which includes the brain and the spinal cord, has almost no ability to repair itself. Unlike the skin and bones, it can’t grow new cells, and damaged cells can’t grow new axons. So when the spinal cord is injured — whether by a motor vehicle accident, a fall, a sports injury or disease — parts of the body are cut off from the brain’s command centers. In severe cases, patients can no longer move or feel below the point of injury.
Evolutionary fork in the road
Why is there so little capacity for regenerating nerve connections? “The general view is that over the course of evolution, there was kind of a tradeoff between maintaining the accuracy of the circuitry and the ability to remain plastic and regrow,” says Larry Benowitz, PhD, a Kirby Center researcher.
The billions of neurons in the central nervous system of each human being make trillions of connections – “more connections than there are stars in the universe,” Woolf notes. And those connections allow us to learn languages, form memories, develop consciousness and more. These qualities are so essential to our survival that natural selection is thought to have favored those ancestors who gave up the ability to grow new neurons in favor of retaining the connections they already had.
From an evolutionary perspective, Woolf says, the wiring of the central nervous system is so precious “that you’ve gotta lock it down and just preserve it. If that means that we can’t recover after injury, so be it.”
The result is that for many of the 50,000 people worldwide who suffer spinal cord injuries each year, the best treatments have been physical rehabilitation therapy, in hopes of regaining some function, and counseling to adjust to a life with paralysis.
The idea of actually repairing the spinal cord was long considered a lost cause. “We were very good at showing that the central nervous system didn’t regenerate,” Woolf says. “And we had no clue how to change it.”
Peripheral work hints at growth trigger
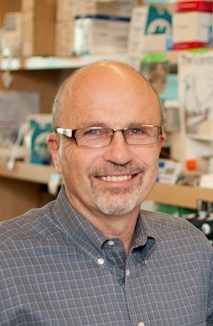
Woolf was so discouraged that he gave up about 20 years ago and turned his attention to the peripheral nervous system — the neurons that connect the spinal cord to the body’s organs and limbs. Unlike those in the central nervous system, peripheral neurons can regrow their axons after injury. Moreover, when injured a second time, their response is faster and more robust. “We were very interested in that, because it hinted to us that there was a regeneration pathway that could be switched on,” Woolf says.
Could that same switch be turned on in the spinal cord? To investigate this idea, Woolf studied an unusual group of spinal cord neurons whose axons split in two, with one branch extending into the peripheral nervous system and the other into the central nervous system. Ordinarily, only the peripheral branch regrows after injury. But Woolf wondered what would happen if he injured the peripheral branches, tipping the cells into a growth state, and then injured the central axons of those cells. “And to our amazement, they regrew,” Woolf says.
This finding undercut the prevailing view that the central nervous system was an implacably hostile environment for the regrowth of nerve cells. The inhibitory factors, though real, could be overcome, Woolf says. “You’ve just got to trigger the cells to enter into a growth state.”
Discovering a hidden potential for repair
Much of the work in the field over the last two decades has focused on how to do that. And the resulting studies at Boston Children’s and elsewhere have begun to unlock the nervous system’s hidden potential for repair.
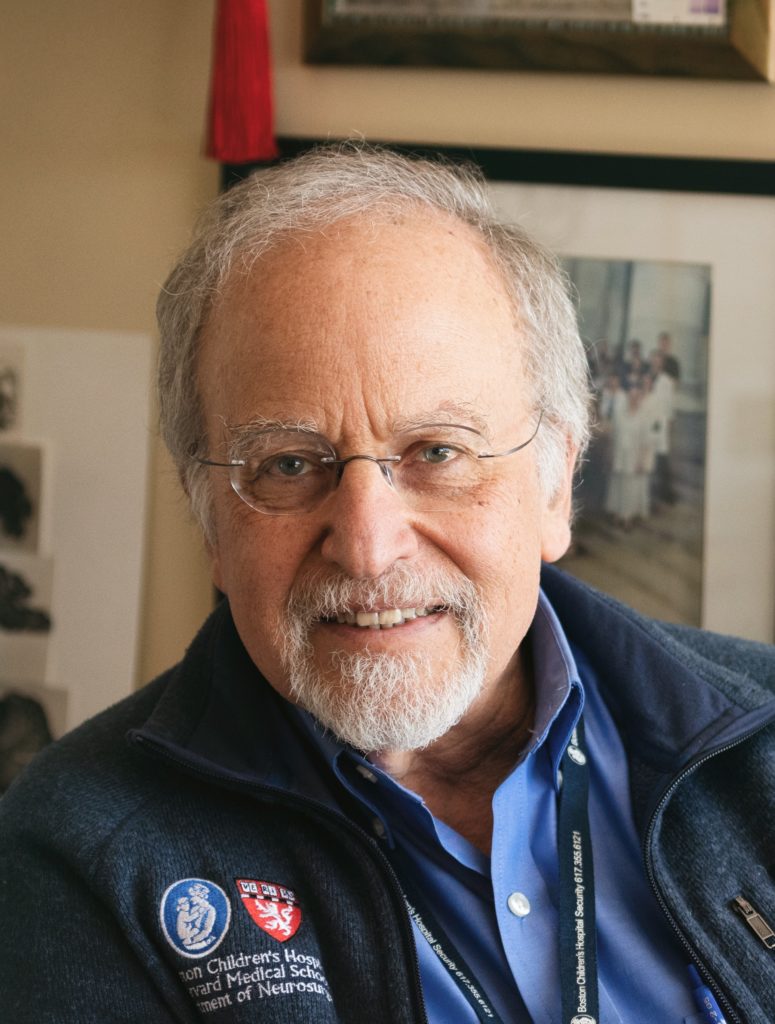
For example, while severed axons cannot grow long distances to form new connections, they can sprout collateral branches. Neuroscientist Martin Schwab, PhD, of the University of Zurich found that those branches form “compensatory circuits,” particularly in response to physical rehabilitation therapy. “The discovery of compensatory circuits was, at least at the basic science level, a real breakthrough,” says Benowitz. “We saw this happen in animal models, and then people started realizing that this occurs to some extent in people.”
Building on Schwab’s work, Benowitz discovered in 2013 that a naturally occurring compound called inosine, given to spinal cord-injured rats, tripled axons’ ability to form collateral branches, creating a “detour circuit” around the site of injury and improving the animals’ use of their limbs.
Stem cells, growth factors and drug cocktails
While scientists at Boston Children’s and elsewhere are seeking ways harness the body’s own powers of repair to treat spinal cord injuries, other scientists are using technology to leapfrog over the injury and re-activate paralyzed limbs.
Neuroscientists like Susan Harkema, PhD, at the University of Louisville have shown that a mild electrical current applied to the base of the spinal cord, below the point of injury, allows paralyzed patients to regain some control of their legs and feet. The effect of this “epidural stimulation” is especially pronounced when combined with physical rehabilitation, with a few patients even regaining the ability to walk with support. Boston Children’s Zhigang He calls it “probably the most exciting advance in the spinal cord injury field in the past ten years.”
At the École Polytechnique Fédérale in Lausanne, Switzerland, neuroscientist Grégoire Courtine, PhD, has gone a step further. Working with partially paralyzed monkeys, he implanted electrodes to record the activity in the part of the brain that directs leg movements, then used a wireless transmitter to send those signals to an electrical stimulator at the base of the monkeys’ spinal cords. The result: When the monkeys think of walking, the pattern of brain electrical activity is reproduced below the site of the spinal cord injury, allowing the monkeys to walk. Courtine has already begun a limited clinical trial of the technology.
At the University of California San Diego, Mark Tuszynski, MD, PhD, and his colleague Paul Lu, PhD, have taken stem cells, induced them to become neuron-like, and implanted them in spinal cord-injured rats. “Even though the rat’s injured neurons cannot extend lengthy axons, the stems cells placed into the injury site form remarkably long connections,” Benowitz says.
At UCLA, neurobiologist Michael Sofroniew, MD, PhD, has used certain proteins to promote regrowth of severed axons. When he filled a gel with these growth factors and placed it below the site of a spinal cord injury in mice, axons grew into it across the injury site. A second gel, placed even lower, induced more growth. “One could imagine, in principle, that you could put these further down the spinal cord and get the axons to keep growing,” Benowitz says.
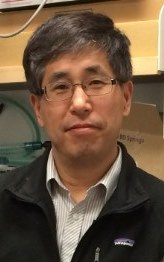
And in 2017, He discovered that a “cocktail” of three compounds, given to mice with a spinal cord injury affecting one side of the body, promoted axon regeneration. More important, the axons made useful new connections to other nerve cells, markedly improving the animals’ ability to climb a ladder. “For the first time, we have a drug treatment that promotes functional recovery after spinal cord injury,” He said at the time.
“Through the kinds of studies that Zhigang and Larry and many others have conducted, we can now manipulate the central nervous system in such a way that we can get real growth,” Woolf says. “That is an enormous breakthrough in the biology of the response to injury and how to change it. We have changed the natural order of things.”
Reawakening dormant neurons
More recently, He has turned his attention to another piece of the puzzle: Most patients with spinal cord injuries suffer damage to only a part of the spinal cord, yet they often suffer from complete paralysis below the point of injury. Why don’t the undamaged fibers keep working, allowing at least some movement?
To find out, He and his colleagues studied mice with partial spinal cord injuries. They found that around the site of the injury, inhibitory neurons — whose role is to tamp down the activity of surrounding cells — are overactive, effectively blocking signals from the brain. But the team also found they could overcome this inhibition by treating the mice with a compound called CLP290. “We saw 80 percent of mice treated with this compound recover their stepping ability,” He said in 2018.
This approach — reawakening dormant but undamaged neurons — is the one He hopes to begin testing on people with spinal cord injuries. He’s been meeting with clinical experts to examine the logistics of starting a human trial. “I’m not sure it will happen,” he says, “but that is my goal. The whole idea is to try to build a bridge to move this finding to the clinical side.”
Through the kinds of studies that Zhigang and Larry and many others have conducted, we can now manipulate the central nervous system in such a way that we can get real growth. That is an enormous breakthrough in the biology of the response to injury and how to change it. We have changed the natural order of things.
No longer an impossible dream
Woolf, Benowitz and He all caution that none of these advances represent an immediate cure for spinal cord injuries. Before the treatments can be used in people, they must be proven safe and effective — in humans as well as animals. But we may soon find out if some of them work. “Everything we’ve learned will be tested in clinical settings in the next ten years,” He predicts.
Woolf is even more optimistic. “We will be able to utilize the non-injured surviving parts of the nervous system in a way that we haven’t before to recover function that has been lost,” he says. “We will also be able to trigger regrowth of damaged neurons. And we’ll be able to cause local sprouting and reestablish new circuits that can help aid the restoration of function.”
“I don’t think it’s going to be immediate,” Woolf says. “But it’s no longer an impossible dream. This is going to be a reality in 10 to 20 years.”
Related Posts :
-
Lucas receives gene therapy for DMD and finds his super muscles
Lucas Toro has a lot in common with Gekko, the cartoon character from PJ Masks. They’re both “little guys” ...
-
Exploring brain operations: Making decisions, snapping to attention, and forming memories
How do our brains snap to attention and orient us to the outside world — like when we’re sound asleep ...
-
The journey to a treatment for hereditary spastic paraplegia
In 2016, Darius Ebrahimi-Fakhari, MD, PhD, a neurology fellow at Boston Children’s Hospital, met two little girls with spasticity and ...
-
It takes a village and the world: Tariq’s care for Tourette syndrome
When your child is sick but you can’t figure out the cause or how to fix it, it can ...