Exploring an unsung part of the brain: the choroid plexus
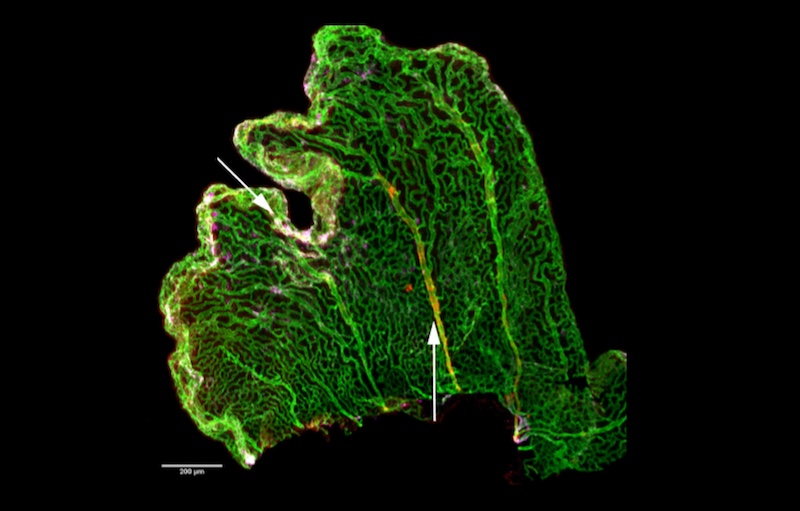
If you’ve never heard of the choroid plexus, you’re not alone. In fact, few neuroscientists know much about this part of the brain. In the words of the late comedian Rodney Dangerfield, the choroid plexus “don’t get no respect.”
But that’s beginning to change, thanks in part to Maria Lehtinen, PhD, who has made the choroid plexus the focus of her research.
“This is really where my heart lies right now,” says Lehtinen, the Hannah C. Kinney Chair in Pediatric Pathology Research at Boston Children’s Hospital.
The choroid plexus consists of four distinct tissues found deep inside the brain. Together, they’re an important source of the cerebrospinal fluid, or CSF, that bathes the brain and spinal cord. The CSF surrounds the brain and circulates inside it through four channels called ventricles. Each ventricle has its own stretch of choroid plexus tissue, anchored like a kelp bed, its pink fronds undulating in the circulating fluid.
Renewed appreciation for a ‘highly gifted juice’
Hundreds of years ago, scientists and philosophers believed the CSF was a “highly gifted juice” that played a vital, dynamic role in the body. But in modern times it came to be regarded as a mere supporting player whose only roles were to cushion the brain and help maintain its proper salt balance.
More recent research, however, has begun to refocus attention on the CSF. In 2011, for example, Lehtinen was a postdoc in the laboratory of Christopher Walsh, MD, PhD, chief of the Division of Genetics and Genomics at Boston Children’s, when they made three discoveries. First, the CSF is filled with hundreds of proteins and growth factors with potential to influence the developing brain; second, its content changes as an organism ages; and third, neural stem cells of the brain do best in age-appropriate CSF.
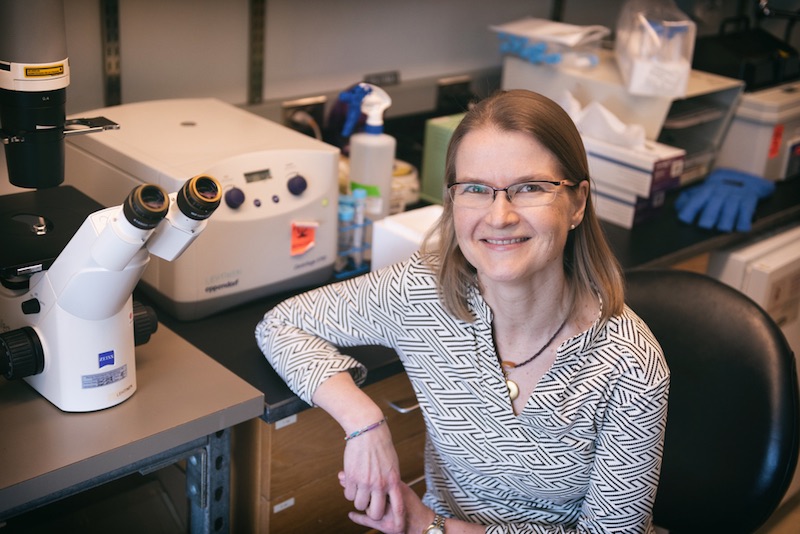
The work was a turning point for Lehtinen. “I started loving the CSF the first day we started thinking about it,” she says. “And I knew that’s what I wanted to work on in my lab.”
Before long, however, Lehtinen turned her attention to the source of the CSF. “It’s very difficult to study just a fluid,” she says. “It’s like batting at the wind. Looking at the choroid plexus at least gives us a tissue and some real cells we can start studying.”
A tissue with many roles
It was more than a century ago that the “father of neurosurgery,” Harvey Cushing at the Peter Bent Brigham Hospital in Boston, and his contemporary, Walter Dandy, determined that the choroid plexus is a key source of CSF. Since then, scientists have discovered other important functions it performs for the brain.
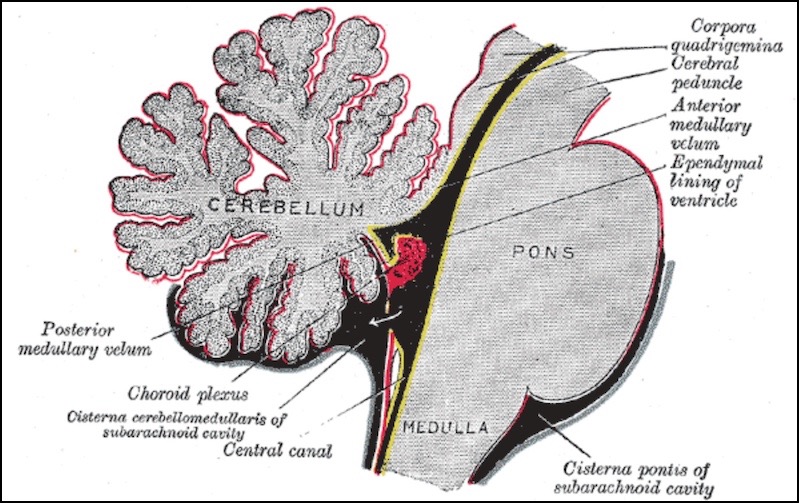
One is basic housekeeping. The brain is, in a sense, cut off from the rest of the body by the “blood-brain barrier” — a boundary that blocks many cells and molecules, as well as pathogens, from entering the brain’s blood supply. The barrier provides vital protection, but it also robs the brain of the blood-cleansing services provided by organs like the kidney and liver.
“In order to deal with that, the choroid plexus looks for imbalances in CSF health and can activate a rapid response to brain emergencies,” says Cameron Sadegh, MD, PhD, who is spending two years doing his postdoctoral research in Lehtinen’s lab.
Sadegh, also a neurosurgery resident at Massachusetts General Hospital, notes that certain cells within the choroid plexus express the same genes that are turned on in kidney and liver cells. And they use some of the same ion channels and enzymes that those other organs use to cleanse the blood.
“When there’s trouble in the brain, the choroid plexus is one of the gatekeepers,” he adds. If the brain sustains an infection, a stroke, or some other injury, for example, immune cells can’t get past the blood-brain barrier to attend to the damage. “Where do they go? Often through the choroid plexus,” Sadegh says. “It lets them in.”
Overshadowed by neurons
Like the CSF, the choroid plexus has been relegated to the back burner for much of the last 40 years, overshadowed by the darling of neuroscientists: nerve cells. “Neurons — that’s where the action is,” Lehtinen says.
There’s good reason to be fascinated with neurons: Their electrical activity conveys our sensations, triggers our thoughts, and controls our movements. But neurons have also enjoyed another advantage: the availability of powerful tools for studying them.
In the late 1970s, the Nobel Prize-winning invention of the patch clamp gave neuroscientists a way to stimulate individual neurons and see how electrical impulses, or action potentials, propagate through the nervous system, affecting the brain and other parts of the body. Then, in the 1980s and 1990s, imaging technologies like MRI and PET scanning allowed scientists to watch the brain in action in real time.
The humble cells of the choroid plexus were no match for that kind of competition. “These are not neurons. They’re not going to spike action potentials,” Lehtinen says. “So the choroid plexus has historically been neglected in neuroscience.”
New tools: mass spec and single-cell sequencing
In the last 15 years, however, new tools have emerged that allow scientists to tackle the choroid plexus in ways they couldn’t before. Applying the technique of mass spectrometry to identify peptides, for example, Lehtinen’s team has been able to reconstruct the proteins contained in the CSF.
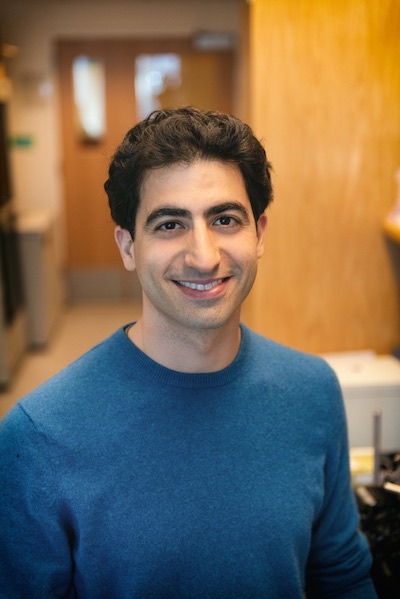
“It’s like if you went to Jamba Juice, and you wanted their recipe, but they wouldn’t give it to you,” explains Sadegh. “We can just run their sample through a mass spec, and it could tell you the distribution of different fruits in that smoothie.”
Another new technology is single-cell sequencing, which allows scientists to isolate individual cells and determine which genes are turned on in them. Building on the work of graduate student Melody Lun, Neil Dani, PhD, a Reagan Sloane Shanley Scholar in Lehtinen’s lab, used this technique in the choroid plexus, together with colleagues at the Broad Institute. They discovered that the genes expressed in the choroid plexus tissue in one ventricle are different from those expressed in a different ventricle — upending the common belief that all four tissues are the same.
“We were humbled very quickly when we realized that each ventricle has its own choroid plexus character,” Lehtinen says. “Now the tools are there, and we can start to understand what the biology of the tissue is.”
Collaboration on hydrocephalus
Another major focus of Lehtinen’s work is a condition called hydrocephalus — a progressive increase of fluid within the ventricles that can damage the brain, enlarge the head, and even lead to death. Sometimes called “water on the brain,” hydrocephalus can be caused by overproduction of CSF by the choroid plexus, a blockage of CSF flow, or failure of the bloodstream to absorb CSF at the same rate it’s being produced.
In infants with hydrocephalus, the excess CSF accumulated within the ventricles can cause brain damage and other problems. For more than 50 years, the standard treatment has been to insert a shunt — a tube that allows excess CSF to drain from the brain into the abdomen, where it’s absorbed by the body. However, shunts often require multiple follow-up surgeries, which are difficult for patients who don’t have easy access to major hospitals.
Lehtinen recently began a collaboration with Boston Children’s neurosurgeon Benjamin Warf, MD, who has pioneered an alternative treatment called endoscopic third ventriculostomy with choroid plexus cauterization (ETV/CPC). It has two parts: 1) making a small hole in one of the ventricles to allow excess fluid to drain out, and 2) burning off part of the choroid plexus to reduce the production of CSF.
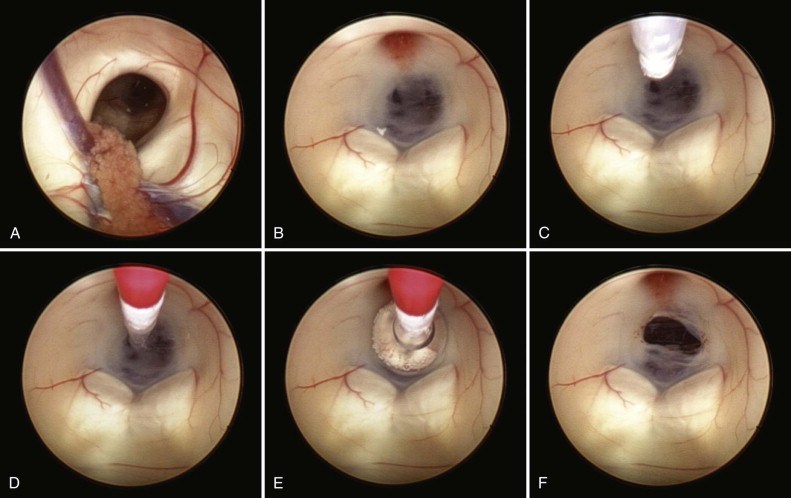
While ETV/CPC can avoid the need for shunts in most children with hydrocephalus, a shunt is often needed if ETV/CPC fails. The collaboration between Warf and Lehtinen aims to understand why a shunt is necessary in some situations, and to help future children, their families, and their care teams choose the most helpful surgery at the very beginning.
Warf and his collaborators Jason Sutin, PhD, and Ivy Lin, PhD, from the Boston Children’s Fetal-Neonatal Neuroimaging and Developmental Science Center, now hope to gather physiological data from several carefully selected children on whom Warf performs ETV/CPC at Boston Children’s.
Meanwhile, Lehtinen and Sadegh are studying how hydrocephalus develops in a mouse model. Huixin Xu, PhD, and Ryann Fame, PhD, on Lehtinen’s team are discovering features of the mouse model that can be used for comparison with patient data.
By sharing their teams’ data, Warf and Lehtinen hope to develop a set of indicators that predict the type and severity of hydrocephalus to guide the best treatment. Their work is powered by long-standing support from the Pediatric Hydrocephalus Foundation, the Hydrocephalus Association, and the New York Stem Cell Foundation, as well as the dedication of patients and their families.
A ‘skylight into the brain’
Lehtinen is also developing new research tools to shed further light on the choroid plexus, including one she calls a “skylight into the brain.” It involves removing the scalp, part of the skull, and the top of the brain in experimental mice, then replacing them with a piece of plexiglass that affords a view directly into the choroid plexus.
The imaging team in the Lehtinen lab can then insert a fluorescent marker to indicate calcium activity in the choroid plexus. The result looks like a thunderstorm in the brain — periodic flashes of light, each one indicating activity in a particular cell.
“Once the window is in place, we can see the entire choroid plexus alive and well in its home environment,” Sadegh says. “We can image any individual cell, and we can also tag a smaller part of the choroid plexus to study how it responds to either normal or harmful factors in the CSF or bloodstream.” Lehtinen and her colleagues were just awarded an NIH Brain Initiative Grant to further advance this technology.
More to the brain than neurons
When Lehtinen set out to study the CSF and the choroid plexus in 2011, hers was a lonely quest in a field preoccupied with neurons. But today the brain’s other components are attracting a growing number of scientists.
One is Boston Children’s Beth Stevens, PhD, who’s made pivotal discoveries about the brain’s glial cells, once also considered to be merely supportive in nature. Stevens has shown that microglia play a key role in pruning the synapses between neurons, thereby shaping brain connections.
Lehtinen is glad to be working in the company of this growing band of scientists. Their motto, as Lehtinen puts it, might be: “There’s a lot more to the brain than neurons.”
The Lehtinen lab and its members are currently supported by the Pediatric Hydrocephalus Foundation, Hydrocephalus Association, Reagan Sloane Shanley Scholarship, William Randolph Hearst Foundation, National Science Foundation, Simons Foundation, Harvard Brain Science Initiative, Human Frontier Science Program, National Institutes of Health, and the New York Stem Cell Foundation. Lehtinen is a New York Stem Cell Foundation – Robertson Investigator.
Related Posts :
-
Phenylketonuria: Giving treatment a second chance
Phenylketonuria (PKU) was once a common cause of intellectual disability. Children born with this metabolic condition lack phenylalanine hydroxylase (PAH), ...
-
Mapping cells to create targeted treatments for interstitial lung disease
John Kennedy, MD, MSc, remembers the relative simplicity of his first genetic mapping project. In a Harvard Medical School&...
-
Changing lives through genetics: The Children’s Rare Disease Collaborative
A 14-year-old girl was having back pain after a car accident and visited an orthopedic clinic at Boston Children’s ...
-
Mutations during prenatal development may contribute to schizophrenia
Schizophrenia is known to have a genetic component, and variants in 10 genes have been identified as markedly increasing schizophrenia risk. ...